Introduction
Nowadays, Inconel 718 is used in jet engine and generator applications because of its high strength and good corrosion resistance. Inconel is a Ni-based superalloy and used for high-temperature applications. However, the formability behavior of Inconel 718 is significantly low when subjected to elevated temperatures. To overcome the low formability at high thermal environment, the forming temperature was increased above the recrystallization temperature for Inconel 718. Many researchers suggested that superplastic behavior was seen when subjected to low-stress conditions (1, 2). Importantly, the primary prerequisite for the advent of superplasticity is the grain size of the material which is typically less than 10 μm (3). This means that the lower the grain size, higher the strain rate for the superplasticity to occur.
Severe plastic deformation (SPD) improved the feasibility of grain refinement. Many SPD processes are available such as surface mechanical attrition treatment, Equal Channel Angular Pressing, and high-pressure Torsion (HPT). But HPT is most significant, for difficult to deform metals or alloys or ceramics and exhibits the ability to refine the microstructure at room temperature (4). The HPT technique has been effectively utilized for intermetallics and ceramics which display better strength and lesser ductility.
In other words, processing the material through mechanical treatment significantly improves the mechanical strength via grain refinement and it leads to modification of the texture of materials. Many researchers focused on the microstructure and mechanical characterization of Inconel 718. The literature on the superplasticity of Inconel 718 through grain refinement is scarce. Hence, the objective of the study is to analyze the superplasticity behavior of Inconel 718 using HPT at various pressures such as 4, 5 and 6 GPa. The grain refinement was analyzed using electron backscattered diffraction (EBSD). The hardness and tensile strength of the Inconel 718 were analyzed using Vickers hardness and Tensile tester. Superplasticity along with elongation to failure was analyzed after the HPT process. The texture surface analysis was performed employing pole figures and orientation distribution function (ODF) graphs.
Methods
In the present research, Inconel 718 specimens having dimensions 20 mm diameter and 0.5 mm thickness was used. At first, the surface of samples were etched with 75% HCl solution to remove any oxide impurities. The HPT technique is well-known for its ability to develop ultrafine-grained microstructures, which drastically modify the mechanical characteristics of materials like hardness, tensile strength, and fatigue resistance. Such improvements are particularly beneficial for applications in aerospace and other high-stress applications where superior mechanical performance is important. Before initiating the HPT process, it was required to ensure that no slippage should occur between the sample and the anvils. Slippage may result in irregular deformation, causing inconsistent mechanical properties within the samples. Strategies were taken to securely position the sample between the anvils, confirming that the applied pressure and rotating forces were efficiently delivered to the sample.
The HPT process was initiated by placing the specimens between two anvils. Input pressure of 4, 5, and 6 GPa was applied at room temperature. During the HPT procedure, simultaneous compression and torsional deformation occurred. A noteworthy observation was made during the HPT process where the material flows around the edge of the disk. This indicates the occurrence of severe deformation within the sample, which is required to enhance the mechanical properties. The anvil’s rotating speed remained at a constant 2 rpm, enabling uniform deformation across the sample. The uniform rotation speed is essential for attaining uniform microstructural refinement.
The microstructural changes that happens within Inconel 718 samples were studied. The SPD induced by the HPT process promotes grain refinement, leading to a more uniform and stable microstructure. This refined microstructure contributes to the improved mechanical properties observed in the samples. The grain refinement of the samples was characterized using EBSD. The pole and ODF figures of the processed samples were assessed for crystallographic texture. Vickers hardness was measured at eight different positions of the polished surface at 500 g load with an interval of 10 seconds and the average values are reported. Samples for the tensile test were cut using electron discharge machining.
Results and discussion
The typical EBSD for the sample subjected to HPT process at 6 GPa is shown in Figure 1. It is observed that the different colors indicate different grain orientations. According to Figure 1, after HPT process, Inconel 718 grain structure was refined as equi-axed grains. In addition to that, smaller strain occurred between the grains and it lead to the formation a bimodal grain size distribution (5).
Figure 2 shows the pole and ODF plot for the HPT-processed sample at 6 GPa. It demonstrates that a regular texture was produced with increasing intensity as strain approached the sample’s edge. The ODF plot (refer Figure 2) further confirms the regular texture development on the samples. Increasing the processing pressure leads to homogenous regular texture on the surfaces. EBSD of the HPT-processed sample at 6 GPa pressure illustrated (Refer Figure 1) furthermore, establishes consistency of the grain orientation throughout the cross-section of the samples. The grain orientation is viewed in two directions; one is perpendicular and the other is parallel to the disk. In the EBSD image, three distinguishing colors have been observed. Strong green reveals the formation of strong regular texture throughout the disk, parallel to the surface. Black and pink indicated that the grain orientation is in an elongated manner (6, 7).
The grain size distribution to the area fraction for HPT-treated specimens (Refer Figure 3a). Both center and edge of the sample surfaces were analyzed. It is evident that for the samples processed at 6 GPa, there is no obvious variation in the grain size distribution for both center and edge of the sample surfaces (5, 8).

Figure 3. (a) Grain size distribution vs. Area fraction and (b) Vickers micro-hardness of the HPT-treated specimens against equivalent strain.
Vickers micro-hardness of the HPT-treated specimens against equivalent strain is shown in Figure 3b. In order to assess the strength attributes of the HPT-processed samples at 4, 5, and 6 GPa, the equivalent strain (ε) was elucidated using the following empirical equation (1)
where N = number of revolutions; r = radius of disk; t = thickness of the sample.
The hardness was increased significantly for the samples at 6 GPa over other samples. It increased from 200 to 235 HV with an ε of ∼30 against 4, 5, and 6 GPa pressures.
The variations of nominal stress against nominal strain after HPT processing for different pressure conditions (4, 5, and 6 GPa) (Refer Figure 4a). It is clear from the figure that the elongation increased and it confirms the superplasticity of the samples. However, the flow behavior of the HPT-processed samples at 4 GPa is quite different from the other samples processed at 5 and 6 GPa. The Nominal stress of the sample at 4 GPa increased to a maximum of 600 MPa before decreasing.
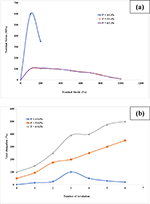
Figure 4. (a) Nominal stress vs Strain for HPT-treated samples and (b) superplasticity along with the elongation to failure with respect to revolution.
The superplasticity along with the elongation to failure is more than 500% and was attained for HPT-processed samples at 6 GPa as shown in Figure 4b. However, this is not true for HPT specimens treated at 4 and 5 GPa, which can be attributed to the inadequate fine grain structure (2, 7).
Conclusion
• HPT effectively treated Inconel 718 alloy at pressures of 4, 5, and 6 GPa.
• Inconel 718, grain structure was refined as equi-axed grains after HPT processing and also the bimodal grain size distribution occurred based on strain.
• ODF plot confirms that increasing processing pressure leads to the formation of homogenous regular texture on the surfaces.
• Superplasticity along with the elongation to failure is more than 500% was attained for the HPT samples processed at 6 GPa.
References
1. Mukhtarov, S, Dudova, N, and Valitov, V. Processing and mechanical properties of bulk nanostructured nickel-based alloys. Mater Sci Eng A. (2009) 503:181–4.
2. Thulasiram, R, Mani, S, Ramaswamy, N, and Murugesan, M. Grain size refinement, texture analysis and effect on the tensile properties of a novel Inconel 718. Mater Lett. (2021) 292:129633.
3. Ahmadkhanih, D, Huang, Y, Jaskari, M, Jar̈venpa, A, Heydarzadeh Sohi, M, Zanella, C, et al. Effect of high-pressure torsion on microstructure, mechanical properties and corrosion resistance of cast pure Mg. J Mater Sci. (2018) 53:16585–97.
4. Straumal, AB, Tsoy, KV, Mazilkin, IA, Nekrasov, AN, and Bryła, K. Grain boundary wetting and material performance in an industrial EZ33A Mg cast Alloyarch. Metall Mater. (2019) 64:869–73.
5. Thulasiram, R, Mani, R, Ramaswamy, N, and Murugesan, M. Wetting phase transition of grain boundaries and material performance of novel Inconel 718. Mater. Lett. (2021) 295:129858.
5. Ramkumar, T, Selvakumar, M, Narayanasamy, P, Ayisha Begam, A, Mathavan, P, and Arun Raj, A. Studies on the structural property, mechanical relationships and corrosion behaviour of Inconel 718 and SS 316L dissimilar joints by TIG welding without using activated flux. J Manuf Process. (2017) 30:290–8.
7. Takizawa, Y, Otsuka, K, Masuda, T, Kajita, T, Yumoto, M, and Otagiri, Y. High-strain rate superplasticity of Inconel 718 through grain refinement by high-pressure torsion. Mater Sci Eng A (2015) 648:178–82.
8. Tang, Y, Sumikawa, K, Takizawa, Y, Yumoto, M, Otagiri, Y, and Horita, Z. Multi-pass high-pressure sliding (MP-HPS) for grain refinement and superplasticity in metallic round rods. Mater Sci Eng A (2019) 748:108–18.
© The Author(s). 2024 Open Access This article is distributed under the terms of the Creative Commons Attribution 4.0 International License (https://creativecommons.org/licenses/by/4.0/), which permits unrestricted use, distribution, and reproduction in any medium, provided you give appropriate credit to the original author(s) and the source, provide a link to the Creative Commons license, and indicate if changes were made.